How does your phone instantly know it’s tilted? Often, it’s the accelerometer at work. This tiny sensor, now in billions of devices globally—from wearables to vehicles—is a cornerstone of modern motion and orientation sensing. Its impact in 2025 is immense, powering countless applications from health tracking to navigation.
We’ll explore the accelerometer’s fundamental functions, its technological significance, and the core concepts of acceleration it so precisely measures, making our digital experiences seamless and intuitive.
Table of Contents
What is an Accelerometer?
An accelerometer is an electromechanical sensor designed to measure acceleration forces. These forces can be static, such as the constant pull of gravity, or dynamic, arising from movement, vibration, or impact. Its primary purpose is to quantify the rate at which an object’s velocity changes or to detect the forces acting upon it. Given their widespread integration, the market for MEMS sensors, which prominently includes accelerometers, is valued at over $15 billion annually in 2025, highlighting their critical role.
Core Functions of an Accelerometer These versatile sensors perform several fundamental functions:
- Measuring Acceleration: They provide quantitative data on changes in speed or direction. Common 3-axis accelerometers offer comprehensive, multi-dimensional sensing capabilities.
- Detecting Motion: Accelerometers can determine if an object is moving and characterize its movement (e.g., shaking, tilting, rotating).
- Sensing Orientation (Tilt): By continuously measuring Earth’s gravitational force, multi-axis accelerometers determine a device’s orientation relative to the ground—effectively identifying “which way is down.” This is crucial for features like screen rotation in the billions of smartphones in use.
- Detecting Vibration: These sensors are adept at sensing oscillatory movements and vibrations within objects or systems, vital for industrial monitoring.
- Detecting Impact: Accelerometers play a key role in identifying sudden shocks, impacts, or collisions, essential for automotive safety systems and protecting portable electronics.
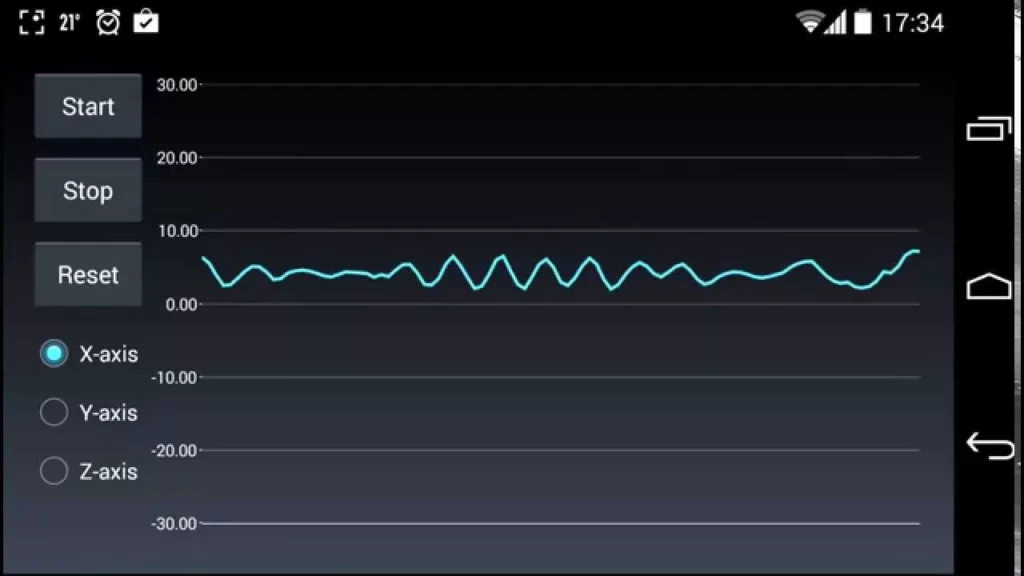
The Significance of Accelerometers in Modern Technology
The integration of accelerometers into modern devices has been transformative, profoundly impacting user experience, safety, and operational efficiency across numerous fields. In 2025, these sensors are fundamental enablers of core functionalities in billions of devices worldwide.
Enabling Interactive User Experiences In consumer electronics, accelerometers are essential for features now integral to our daily interactions.
- Smart Devices: Automatic screen rotation in smartphones and tablets is a primary example.
- Gaming: Motion-controlled gaming, a market segment valued at well over $100 billion annually, heavily relies on accelerometer data.
- Fitness Tracking: The step-counting and activity recognition capabilities of fitness trackers and smartwatches are powered by these sensors, helping millions monitor their health.
Enhancing Safety and Efficiency Beyond consumer gadgets, accelerometers are critical components where safety and efficiency are paramount.
- Automotive Safety: They are vital for triggering airbag deployment during collisions—a technology credited with saving tens of thousands of lives annually—and are integral to anti-lock braking systems (ABS) and electronic stability control.
- Industrial Applications: Accelerometers enable predictive maintenance by monitoring machinery vibrations for early signs of wear, which can reduce unplanned downtime by an estimated 30-40% in some industries. They are also used in structural health monitoring for buildings and bridges.
The MEMS Revolution: Miniaturization and Cost-Effectiveness A key factor in the widespread adoption of accelerometers has been Micro-Electro-Mechanical Systems (MEMS) technology. MEMS has enabled the production of accelerometers that are:
- Incredibly Small: Allowing integration into compact devices.
- Power-Efficient: Crucial for battery-operated portable electronics.
- Cost-Effective: Making mass deployment economically viable. This technological leap has fueled the multi-billion dollar MEMS sensor market (valued at over $15 billion annually), with accelerometers being a key component. It has made sophisticated motion-sensing feasible across a broad spectrum of devices, especially in mobile and consumer markets.
Leveraging Accelerometer Data: Core Applications and Use Cases
Accelerometer data, representing forces and movements, is the raw material for a multitude of applications enhancing device functionality, user interaction, and safety across the technological landscape of 2025.
3.1 Device Orientation and Screen Rotation One of the most familiar applications in mobile devices, ubiquitous in the billions of smartphones and tablets used globally.
- Mechanism: Multi-axis accelerometers continuously sense Earth’s gravitational pull, determining “which way is down” relative to the device. By analyzing gravity’s components along its axes, an application discerns if the device is in portrait, landscape, or another angle.
- User Benefit: Automatically adjusts display content to match viewing orientation, enhancing usability without manual adjustments.
3.2 Motion and Gesture Detection Accelerometers adeptly detect various physical movements and gestures.
- Basic Gestures: Readily identifies tilts (static acceleration changes), shakes (rapid acceleration changes), taps/double taps (short, sharp impacts), and swings.
- Application Examples: These trigger actions like controlling games by tilting, shaking to undo or shuffle music, or tapping to wake a device.
- Advanced Gesture Recognition: Beyond simple movements, nuanced gestures are recognized by analyzing acceleration patterns over time, often using algorithms like Dynamic Time Warping or machine learning models for a richer interpretation of user intent.
3.3 Activity and Fitness Tracking Accelerometers are central to modern fitness trackers and health-monitoring applications. The wearable fitness tracker market, heavily reliant on accelerometers, is a multi-billion dollar industry, with hundreds of millions of units shipped annually.
- Step Counting (Pedometer): Analyzes rhythmic acceleration patterns during walking or running to estimate steps, often with high accuracy (modern wearables typically achieve +/- 5% for step counting).
- Workout Detection & Intensity: Identifies various physical activities (walking, running, cycling) by their unique acceleration signatures and estimates workout intensity.
- Sleep Monitoring: Uses movement detection during sleep to infer sleep stages and quality, often more accurately when combined with other physiological sensors.
3.4 Gaming and Interactive Controls Accelerometers provide intuitive and engaging interaction in gaming.
- Motion Input: Players control game elements by physically tilting, shaking, or moving the device (e.g., steering a car in a racing game). The mobile gaming segment, where such controls are prevalent, accounts for over 50% of the total gaming market revenue.
- Immersive Experiences: Direct translation of physical actions into the virtual world enhances player immersion.
3.5 Navigation and Dead Reckoning Accelerometers are key in navigation systems, often as part of Inertial Measurement Units (IMUs).
- Dead Reckoning: By integrating acceleration data over time, it estimates changes in velocity and position, allowing a device to track movement when external signals like GPS are unavailable (e.g., indoors, tunnels).
- Complementing GPS: Refines location accuracy and provides smoother navigation when GPS signals are weak or intermittent.
3.6 Specialized Applications Utility extends far beyond consumer electronics:
- Automotive: Critical for safety.
- Airbag Deployment: Detects rapid deceleration in crashes to trigger airbags in milliseconds, a feature in millions of vehicles.
- Electronic Stability Control (ESC) & Anti-lock Braking Systems (ABS): Provide vehicle dynamics data. ESC systems, reliant on accelerometers, are estimated to reduce fatal single-vehicle crashes by over 30%.
- Vehicle Telematics: Monitors driving behavior for fleet management and insurance.
- Industrial:
- Predictive Maintenance: Monitors machinery vibration to detect early wear, potentially reducing unplanned downtime by 25-50%.
- Structural Health Monitoring: Assesses integrity of bridges and buildings by measuring vibrations.
- Medical/Healthcare:
- Patient Monitoring: Detects falls in at-risk individuals—a critical application as global elderly populations grow. Monitors physical activity for health and rehabilitation.
- Analyzing Human Movement: Used in biomechanics to analyze gait and posture.
- Laptops/Hard Drives: Free-fall detection to park HDD heads and protect against impact damage.
- Aerospace: Essential for navigation, flight control, and structural integrity monitoring.
3.7 Emerging and Unconventional Uses Increasing sensitivity leads to novel applications, some with privacy considerations. Research in this area is active, with dozens of papers published annually exploring new sensor data uses.
- Inferring Physiological Data: Highly sensitive accelerometers may estimate heart and breathing rates by detecting subtle body movements.
- Location Inference via Vibration Patterns: Correlating similar vibration patterns between devices (e.g., on the same vehicle) could infer co-location even without GPS.
- Acoustic Eavesdropping: Potentially reconstructing sound by analyzing minute device vibrations caused by sound waves, even without microphone access.
The advanced sensitivity enabling these innovations also raises privacy concerns if sensor data access is broad or non-transparent. This underscores a growing need for user awareness, transparent app behavior, and robust OS-level controls to balance utility with individual privacy, alongside an ethical responsibility for developers.
The following table summarizes how accelerometer data is interpreted for several common applications:
Common Accelerometer Applications and Data Interpretation
Application/Use Case | Key Accelerometer Data Characteristic | Typical Axes Involved | Brief Description of Interpretation Logic |
Screen Rotation | Direction of static gravity vector. | All 3 (X, Y, Z) | Determine which axis aligns most closely with gravity (or is anti-parallel) to infer portrait/landscape orientation. 4 |
Step Counting | Rhythmic peaks/patterns in acceleration magnitude or specific axes. | Primarily Z-axis (vertical) or vector magnitude of all 3. | Filter data, detect periodic peaks exceeding a threshold, apply timing constraints to validate steps. 12 |
Shake Detection | Sudden, high-magnitude, often repeated changes in acceleration across axes. | All 3 (X, Y, Z) | Calculate rate of change or force; if it exceeds a threshold for a brief period, a shake is registered. Often includes a refractory period. 17 |
Free-Fall Detection | Near-zero acceleration readings on all axes simultaneously. | All 3 (X, Y, Z) | If magnitude of acceleration on X, Y, and Z axes drops below a very low threshold (e.g., <0.3g) for a defined duration (e.g., >20ms), free-fall is detected. 18 |
Basic Tilt Sensing | Static X, Y, Z components of acceleration relative to gravity. | X, Y (for pitch/roll, assuming Z is vertical reference) | Use trigonometric functions (e.g., arctangent) on the ratios of gravity components along different axes to calculate tilt angles (pitch and roll). 3 |
Impact Detection | Sharp, high-magnitude spike in acceleration. | All 3 (X, Y, Z) | If the magnitude of acceleration exceeds a high threshold very rapidly, an impact is detected. 2 |
Vibration Monitoring | Oscillatory patterns in acceleration data. | One or more axes, depending on vibration direction. | Analyze frequency spectrum (e.g., using FFT) and amplitude of acceleration signals to characterize vibrations. 2 |
Practical Examples: Simple Accelerometer App Concepts
With an understanding of how to access and process accelerometer data, you can build a variety of simple yet engaging applications. Many basic detection algorithms rely on thresholding—comparing sensor data against empirically determined values—often combined with time-based conditions to refine accuracy. Here are some practical examples for 2025:
5.1 Shake Detection
A common feature for actions like undoing or shuffling content.
- Algorithm Concept:
- Continuously monitor accelerometer readings (X, Y, Z axes).
- Calculate a metric for acceleration change. A simple method is to sum the absolute differences between current and previous values for each axis: force = |x_current – x_previous| + |y_current – y_previous| + |z_current – z_previous|.
- If this force exceeds a predefined threshold, a potential shake is registered.
- Implement an interval (refractory period) after a confirmed shake to prevent a single continuous movement from triggering multiple events.
- Thresholding: This value is key for sensitivity (higher threshold = more vigorous shake needed) and often requires empirical tuning for the device and desired user experience.
- Implementation Notes:
- Android: Use SensorEventListener for raw accelerometer data.
- iOS: UIResponder class can directly handle shake gestures (UIEvent.EventSubtype.motionShake) via motionBegan(_:with:) and motionEnded(_:with:), simplifying implementation. For custom logic, CMMotionManager provides raw data.
- Cross-Platform (Flutter/React Native): Sensor packages (e.g., sensors_plus for Flutter) or hooks (e.g., useAnimatedSensor in React Native Reanimated) provide data for your detection logic.
- Example Use Cases: Undo typing, shuffle songs, trigger game actions, refresh content.
5.2 Tilt Sensing / Basic Spirit Level
Demonstrates measuring tilt angles relative to gravity.
- Algorithm Concept:
- Obtain static or low-pass filtered accelerometer readings (Ax, Ay, Az) to get a stable gravity vector representation.
- Calculate pitch (rotation around device’s X-axis) and roll (rotation around Y-axis) using trigonometry. For example: pitch = atan2(Ay, sqrt(Ax² + Az²)) roll = atan2(-Ax, Az) (Note: Exact formulas can vary with axis definitions. atan2(y,x) is generally preferred over atan(y/x).)
- Convert results from radians to degrees: degrees = radians × (180 / π).
- Limitations:
- Accuracy is affected by non-gravitational accelerations (movement, vibration).
- Cannot determine yaw (rotation around Z-axis); this requires a magnetometer or gyroscope.
- Single-axis tilt sensitivity decreases significantly near ±90°.
- Implementation Notes: On Android, this involves processing Sensor.TYPE_ACCELEROMETER data and applying these calculations.
- Example Use Cases: Simple spirit level tools, automatic screen orientation, basic tilt-based game controls.
5.3 Free-Fall Detection
Critical for features like hard drive protection or emergency alerts.
- Algorithm Concept:
- During free fall, an object accelerates downwards at ~9.8m/s², so accelerometer readings on all three axes approach zero relative to its own frame.
- Define a low acceleration threshold (e.g., illustrative values range from 0.05g to 0.35g, or roughly 0.5m/s² to 3.4m/s²).
- If acceleration on all three axes (|Ax|, |Ay|, |Az|) simultaneously drops below this threshold and stays there for a short duration (e.g., 20-30 milliseconds), a free-fall event is triggered.
- Thresholds and Duration: Require empirical tuning. A very low threshold (e.g., around 50mg or 0.05g) might be better in some contexts than higher ones to avoid false positives from quick movements.
- Implementation Notes: Some accelerometer chips (e.g., types like LIS3DH) offer hardware free-fall detection. Software versions involve reading sensor data in a loop and checking the threshold/duration conditions.
- Example Use Cases: Protecting HDDs by parking heads, fall detection systems for elderly care, emergency alerts in safety equipment.
5.4 Basic Step Counting
Popular for fitness tracking.
- Algorithm Concept (Peak Detection):
- Often uses the vertical acceleration component or the magnitude of the total acceleration vector.
- Filter raw data (e.g., a band-pass filter for 0.25 Hz to 2.5 Hz, characteristic of human walking/running, or a low-pass filter) to smooth the signal.
- Identify significant peaks (local maxima) in the filtered signal, representing foot strikes.
- Count a step if a peak’s magnitude exceeds a (possibly dynamic) threshold.
- Use windowing and debouncing (e.g., minimum time since last step) to avoid multiple counts for a single step. Some algorithms require a few initial “potential steps” before confirming actual counting.
- Implementation Notes:
- Cross-Platform (Flutter): Packages like sensors_plus give raw data for custom algorithms, while pedometer or step_counter may offer pre-built functionality.
- Native (Android/iOS): Both platforms provide high-level APIs (e.g., Sensor.TYPE_STEP_COUNTER on Android, Core Motion pedometer events on iOS). These are generally recommended for accuracy (often achieving over 95% in controlled conditions) and battery efficiency.
- Example Use Cases: Fitness tracking apps, health monitoring, activity logging.
These examples show how raw accelerometer readings (X, Y, Z values) are transformed into meaningful, human-understandable events through algorithms, filtering, and application-specific logic. The sensor provides the data; the software creates the “intelligent” behavior.
Conclusion
Once specialized, accelerometers are now essential in billions of devices daily, powering countless features in 2025 thanks to MEMS technology. Understanding their data—from static gravity to dynamic motion—is key. Transforming these raw readings into smart app functions, from gesture control to activity tracking, requires expert interpretation and clever software.
With ongoing advancements in sensor tech and edge AI, the opportunities for innovation are immense. Ready to harness this potential? Let us help you develop a cutting-edge accelerometer app solution.